Zero energy projects represent the pinnacle of sustainable construction, delivering buildings that produce as much energy as they consume over the course of a year. As climate change intensifies and energy costs soar, these groundbreaking zero energy buildings have evolved from experimental concepts to achievable, cost-effective solutions for forward-thinking developers and construction professionals.
The integration of advanced building envelope technologies, renewable energy systems, and intelligent energy management platforms has transformed the commercial construction landscape. Industry leaders now recognize that achieving net-zero performance isn’t just an environmental imperative—it’s a compelling business case that delivers long-term operational savings, enhanced occupant comfort, and increased property values.
For construction professionals and project stakeholders, understanding the technical foundations, financial implications, and implementation strategies of zero energy projects has become essential. This comprehensive analysis explores the critical components, best practices, and proven methodologies that drive successful zero energy developments, providing actionable insights for industry practitioners ready to embrace the future of sustainable construction.
Understanding Zero Energy Projects
Core Components of Zero Energy Design
Successful zero energy projects rely on a strategic combination of essential building elements that work together to minimize energy consumption. At the heart of energy-efficient building design lies three critical components: superior insulation, airtight construction, and high-performance systems.
The building envelope must incorporate continuous insulation with high R-values, particularly in the roof, walls, and foundation. This thermal barrier significantly reduces heat transfer and maintains consistent indoor temperatures. Advanced air-sealing techniques, including proper membrane installation and detailed joint sealing, are crucial for achieving the required air changes per hour (ACH) rate of 0.6 or less.
Mechanical systems must be carefully sized and selected, with emphasis on high-efficiency heat pumps for heating and cooling, energy recovery ventilators (ERVs) for air quality, and solar thermal or heat pump water heaters for domestic hot water. LED lighting, Energy Star-rated appliances, and smart controls further optimize the building’s performance while maintaining occupant comfort and functionality.
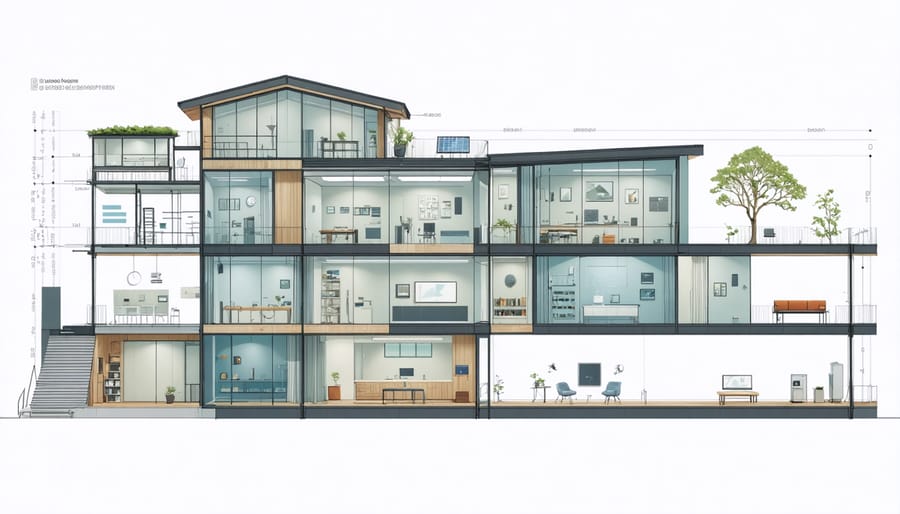
Renewable Energy Integration Strategies
Successful integration of renewable energy systems requires a strategic approach combining multiple technologies and smart implementation methods. Solar photovoltaic (PV) systems typically form the cornerstone of zero energy projects, with roof-mounted arrays being the most common configuration. However, building-integrated photovoltaics (BIPV) are gaining traction, incorporating solar cells into building materials like glass facades and roof tiles.
Wind energy systems, while less common in urban settings, can provide complementary power generation in suitable locations. Ground-source heat pumps leverage stable underground temperatures for efficient heating and cooling, reducing overall energy demand. Energy storage solutions, particularly lithium-ion batteries, are crucial for managing intermittent renewable generation and ensuring consistent power supply.
Smart energy management systems optimize the interaction between these various components, using advanced algorithms to balance generation, storage, and consumption. These systems typically incorporate real-time monitoring and automated controls to maximize efficiency. The key to successful integration lies in proper sizing of components, consideration of local climate conditions, and careful analysis of building energy loads to ensure optimal system performance throughout the year.
Technical Implementation
Building Envelope Optimization
The building envelope serves as the primary defense against energy loss in zero energy projects, making its optimization crucial for achieving maximum efficiency. Advanced materials and construction techniques have revolutionized how we approach envelope design, with significant improvements in thermal resistance and air barrier systems.
High-performance insulation materials, such as vacuum insulated panels (VIPs) and aerogel-based products, deliver superior R-values while maintaining minimal thickness. These materials, combined with strategic implementation of continuous insulation systems, effectively eliminate thermal bridges that traditionally compromise building performance.
Advanced glazing technologies, including triple-pane windows with low-e coatings and gas fills, contribute significantly to envelope performance. Dynamic glazing systems that respond to environmental conditions can further optimize solar heat gain and daylighting throughout the year.
Air-tightness remains paramount, with modern construction techniques emphasizing meticulous detailing at joints and penetrations. Implementation of fluid-applied air barriers and sophisticated sealing systems ensures infiltration rates below 0.6 air changes per hour at 50 Pascals, meeting Passive House standards.
Emerging technologies in prefabricated envelope systems offer precision-engineered solutions that combine multiple performance aspects into single components. These systems not only enhance thermal performance but also reduce construction time and potential installation errors.
Regular thermal imaging and blower door testing during construction verify envelope integrity, while building information modeling (BIM) helps optimize design decisions before implementation, ensuring maximum effectiveness of the chosen materials and systems.
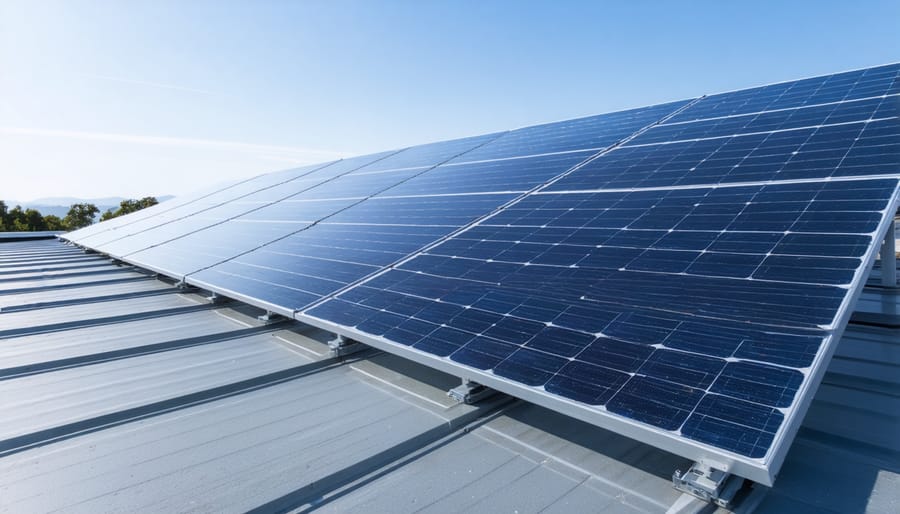
Energy Generation Systems
Renewable energy systems form the backbone of zero energy projects, with solar power generation systems leading the way in both commercial and residential applications. Modern photovoltaic arrays achieve efficiency ratings of 18-22%, utilizing monocrystalline or polycrystalline panels coupled with smart inverter technology to maximize energy capture throughout the day.
Wind energy systems serve as excellent complementary power sources, particularly in regions with average wind speeds exceeding 12 mph. Vertical axis wind turbines (VAWTs) have gained popularity in urban settings due to their compact footprint and ability to harness turbulent wind conditions, while horizontal axis turbines remain the standard for larger installations.
Geothermal heat pumps leverage stable underground temperatures to provide both heating and cooling, achieving coefficients of performance (COP) ranging from 3.0 to 5.0. These systems can reduce HVAC energy consumption by up to 70% compared to conventional systems.
Energy storage solutions, particularly lithium-ion battery systems, play a crucial role in managing power distribution. Modern battery systems offer round-trip efficiencies exceeding 90% and can be scaled from 10kWh for residential applications to several MWh for commercial projects. Integration of smart energy management systems ensures optimal utilization of generated power while maintaining grid stability during peak demand periods.
Smart Energy Management
Smart energy management systems serve as the central nervous system of zero energy projects, continuously monitoring, analyzing, and optimizing energy consumption patterns. These sophisticated systems integrate multiple components, including smart meters, sensors, and automated controls, to create a cohesive network that maximizes energy efficiency.
At the core of these systems are Building Management Systems (BMS) that collect real-time data on energy usage, occupancy patterns, and environmental conditions. Advanced algorithms process this information to make intelligent decisions about heating, cooling, lighting, and ventilation adjustments. For instance, occupancy sensors can automatically modify HVAC settings in unused spaces, while daylight harvesting systems adjust artificial lighting based on natural light availability.
Energy monitoring dashboards provide facility managers with detailed insights into consumption patterns, enabling quick identification of inefficiencies and opportunities for optimization. These platforms typically feature customizable alerts, performance analytics, and predictive maintenance capabilities to prevent energy waste and system failures.
The integration of IoT devices further enhances system capabilities, allowing for remote monitoring and control through mobile applications. This connectivity enables immediate response to changing conditions and automated implementation of energy-saving strategies. Some systems also incorporate machine learning algorithms that adapt to usage patterns over time, continuously improving efficiency without compromising occupant comfort.
To ensure optimal performance, these management systems require regular calibration and updates, with proper training for facility personnel being crucial for maximizing their potential.
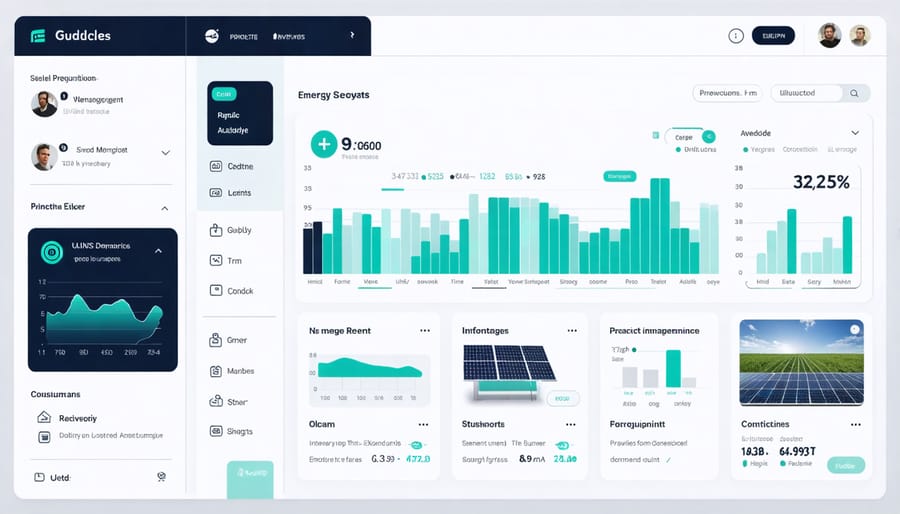
Cost Considerations and ROI
Initial Investment Analysis
The initial investment for a zero energy project typically requires careful financial planning and consideration of multiple cost components. Current industry data indicates that zero energy buildings command a premium of 5-15% above conventional construction costs, depending on project scale and complexity. This investment encompasses high-performance building envelope materials, advanced HVAC systems, renewable energy installations, and smart building controls.
Financing options have evolved to accommodate these specialized projects. Commercial property owners can access green building loans, energy-efficient mortgages, and performance-based financing. Federal and state incentives, including tax credits and grants, can offset 20-30% of initial costs. Power purchase agreements (PPAs) offer an alternative financing structure, allowing organizations to implement renewable energy systems with minimal upfront investment.
A comprehensive cost analysis should consider both hard and soft costs. Hard costs include construction materials, equipment, and labor, while soft costs encompass design, engineering, permits, and commissioning. Industry best practices recommend allocating 60-70% of the budget to hard costs and 30-40% to soft costs, with an additional 10% contingency for unforeseen expenses.
Long-term Benefits
Zero energy projects deliver substantial long-term financial benefits that extend far beyond initial construction costs. Studies show that operational expenses decrease dramatically, with building owners reporting 60-80% reduction in energy costs compared to conventional structures. These energy-efficient investment returns typically materialize within 5-10 years of implementation.
Property values for zero energy buildings consistently outperform traditional structures, showing an average premium of 7-10% in market value. This appreciation is particularly pronounced in commercial real estate, where tenants increasingly prioritize sustainable facilities. Operating costs predictability, achieved through renewable energy systems and advanced building controls, provides a significant competitive advantage in the real estate market.
Furthermore, zero energy buildings demonstrate remarkable resilience against future energy price fluctuations and regulatory changes. As carbon pricing and environmental regulations become more stringent, these properties maintain their market position while conventional buildings may require costly retrofits to meet new standards.
Case Study: Successful Zero Energy Implementation
The Bullitt Center in Seattle, Washington, stands as a landmark achievement in zero energy implementation, demonstrating the practical feasibility of net-zero energy buildings in an urban environment. Completed in 2013, this six-story, 52,000-square-foot commercial building is widely recognized as the world’s first certified Living Building, consistently producing more energy than it consumes.
The project’s success stems from its comprehensive approach to energy efficiency and renewable energy generation. The building features a 242-kW rooftop solar array, comprising 575 photovoltaic panels that generate approximately 60% more electricity than the building uses annually. This overproduction accounts for Seattle’s predominantly cloudy climate, ensuring year-round energy sufficiency.
Key design elements contributing to the building’s exceptional performance include triple-paned windows, automated external blinds that adjust based on solar exposure, and a highly insulated building envelope with an R-value of R-40 for walls and R-60 for the roof. The structure maximizes natural daylight through strategic window placement and light shelves, reducing artificial lighting needs by 80% compared to conventional office buildings.
The mechanical systems showcase innovative solutions, including a ground-source heat pump system with 26 wells drilled 400 feet deep. This system, coupled with radiant floors and ceiling panels, maintains comfortable temperatures while consuming minimal energy. The building’s plug load management system automatically shuts down non-essential equipment during off-hours, resulting in a 75% reduction in plug load energy consumption compared to typical office buildings.
Performance data from the first five years of operation validates the project’s success. The building consistently generates 60% more energy than it uses, with an Energy Use Intensity (EUI) of just 12 kBtu/sf/year – compared to the national average of 93 kBtu/sf/year for office buildings. The project achieved a return on investment within seven years, primarily through energy cost savings and increased tenant satisfaction and retention rates.
The Bullitt Center demonstrates that zero energy buildings can be both technically and financially viable while maintaining high standards of occupant comfort and functionality. Its success has influenced building codes and inspired similar projects across North America, proving that zero energy construction is ready for mainstream adoption in the commercial sector.
Zero energy projects represent a pivotal shift in the construction industry’s approach to sustainable development. As demonstrated throughout this analysis, these initiatives combine advanced building technologies, renewable energy systems, and innovative design strategies to achieve net-zero energy consumption. The successful implementation of such projects requires careful planning, interdisciplinary collaboration, and a thorough understanding of both technical requirements and economic implications.
Looking ahead, the future of zero energy projects appears increasingly promising. Market trends indicate growing adoption rates across commercial, residential, and institutional sectors, driven by stricter energy codes, environmental regulations, and rising energy costs. Technological advancements in building materials, energy storage solutions, and smart building systems continue to make zero energy goals more achievable and cost-effective.
However, challenges remain, particularly in terms of initial investment costs and technical complexity. The industry must focus on developing standardized approaches, improving workforce training, and creating more efficient financing mechanisms to accelerate adoption. Additionally, the integration of emerging technologies such as artificial intelligence and IoT solutions will play a crucial role in optimizing building performance and energy management.
As climate change concerns intensify and energy efficiency becomes increasingly critical, zero energy projects will likely become the standard rather than the exception in construction. Success will depend on continued innovation, policy support, and industry-wide commitment to sustainable building practices.