By 2030, smart cities will fundamentally transform how 68% of the global population lives, works, and interacts with urban infrastructure. These intelligent urban ecosystems integrate advanced IoT sensors, AI-driven systems, and sustainable energy solutions to create unprecedented efficiency and livability. From autonomous transportation networks that reduce commute times by 50% to smart grids that optimize energy consumption in real-time, the smart city revolution represents a $2.5 trillion market opportunity.
Leading metropolitan areas like Singapore, Barcelona, and Copenhagen are already demonstrating how integrated digital infrastructure can reduce carbon emissions by 40%, lower energy costs by 35%, and improve emergency response times by 20-35%. These early adopters provide a crucial roadmap for the widespread implementation of smart city technologies over the next decade.
As we approach 2030, the convergence of 5G networks, edge computing, and renewable energy systems will enable cities to process over 1 petabyte of data daily, delivering actionable insights that enhance everything from waste management to public safety. For industry professionals and urban planners, understanding these transformative technologies and their implementation strategies is no longer optional—it’s imperative for creating resilient, sustainable urban environments that serve the needs of future generations.

The Foundation of 2030’s Smart Energy Grid
Advanced Microgrids and District Energy Systems
By 2030, decentralized energy distribution networks will form the backbone of smart city infrastructure, revolutionizing how urban areas manage and consume energy. Advanced microgrids integrate renewable energy sources, energy storage systems, and intelligent control mechanisms to create resilient power networks that can operate independently or in conjunction with the main grid.
District energy systems optimize energy distribution by centralizing heating and cooling production for multiple buildings, reducing operational costs by 30-50% compared to individual building systems. These networks utilize waste heat recovery, thermal storage, and smart load management to achieve unprecedented efficiency levels.
Recent implementations in Copenhagen and Singapore demonstrate the effectiveness of these systems, with Copenhagen’s district heating network serving 98% of households while reducing carbon emissions by 70%. Integration of AI-powered predictive maintenance and real-time load balancing ensures optimal performance while minimizing downtime.
The economic benefits are substantial, with smart microgrids showing potential ROI within 5-7 years through reduced energy costs and improved grid reliability. Cities implementing these systems report enhanced resilience during extreme weather events and grid disruptions, maintaining critical services when traditional power systems fail.
AI-Powered Energy Management Platforms
In the evolving landscape of smart cities, AI-powered energy optimization systems are revolutionizing how urban environments manage and distribute power resources. These sophisticated platforms utilize machine learning algorithms to analyze vast amounts of real-time data from multiple sources, including smart meters, weather stations, and building management systems.
The integration of AI-driven predictive analytics enables cities to forecast energy demand patterns with unprecedented accuracy, allowing for dynamic load balancing and automated grid management. These systems can reduce peak demand by up to 25% through intelligent load shifting and demand response programs, while maintaining optimal service levels for residents and businesses.
Advanced neural networks continuously monitor and adjust energy distribution based on factors such as occupancy patterns, weather conditions, and grid stability. This results in significant improvements in energy efficiency and substantial cost savings for municipalities. Case studies from pilot programs in Copenhagen and Singapore demonstrate that these AI platforms can achieve energy consumption reductions of 15-20% across entire districts.
Furthermore, these systems facilitate the seamless integration of renewable energy sources by predicting solar and wind power generation capabilities and automatically adjusting grid operations to accommodate fluctuating supply. This capability is crucial for cities working toward carbon neutrality goals, as it enables the effective management of intermittent renewable energy sources while maintaining grid stability.
Renewable Integration and Storage Solutions
Urban Solar Infrastructure
Building-integrated photovoltaics (BIPV) are revolutionizing how smart cities harness solar energy, seamlessly incorporating power generation into architectural elements. Modern facades now feature transparent solar cells that maintain natural lighting while generating electricity, and solar tiles have evolved to match traditional roofing materials in both appearance and durability.
Leading architects are implementing BIPV solutions that achieve up to 40% greater energy efficiency compared to traditional solar installations. These systems integrate with building management platforms, enabling real-time monitoring and optimization of energy production and consumption patterns.
Solar street furniture represents another crucial component of urban solar infrastructure. Smart benches equipped with solar panels not only provide charging stations for mobile devices but also power LED lighting and environmental sensors. Solar-powered waste compactors are reducing collection frequencies by up to 80%, while solar bus shelters generate enough electricity to power digital displays and USB charging ports.
Recent implementations in Copenhagen and Singapore demonstrate how solar infrastructure can be scaled effectively. Copenhagen’s solar-powered smart poles combine 5G connectivity, environmental monitoring, and EV charging capabilities. Singapore’s Gardens by the Bay features solar trees that generate enough power to maintain the complex’s evening light shows while feeding excess energy back to the grid.
These innovations are setting new standards for sustainable urban development, with projected ROI periods decreasing from 15 years to 7-8 years due to improved technology and reduced manufacturing costs.
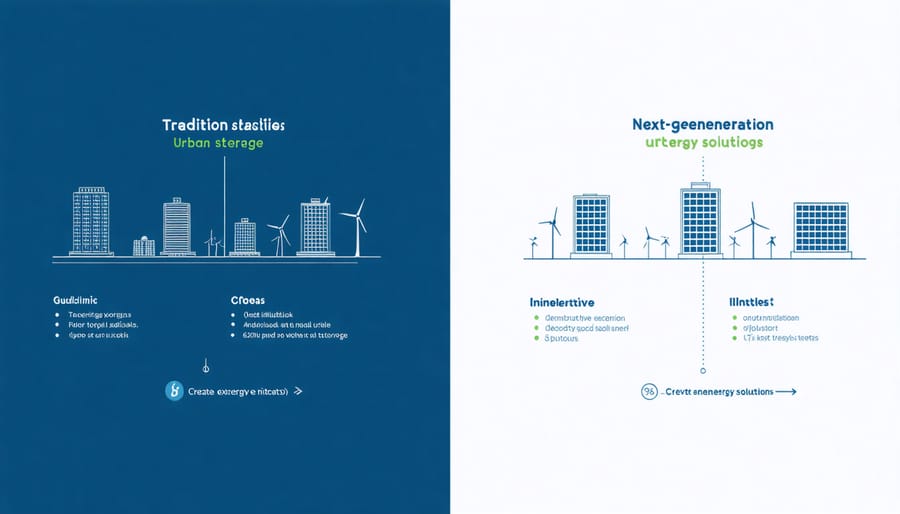
Next-Generation Energy Storage Systems
Next-generation energy storage systems form the backbone of 2030’s smart city infrastructure, combining advanced battery technologies with innovative thermal storage solutions. Grid-scale lithium-ion battery installations are being enhanced with solid-state technology, offering higher energy density and improved safety profiles. These systems can store up to 1,000 MWh of power, enabling cities to effectively manage renewable energy integration.
Thermal energy storage (TES) systems are revolutionizing district heating and cooling networks. Using phase-change materials and molten salt technologies, these systems can store excess heat from industrial processes or solar thermal installations for extended periods. Major implementations in Copenhagen and Dubai have demonstrated energy cost reductions of up to 40% while significantly reducing peak load demands.
Flow batteries are emerging as a promising solution for long-duration storage needs, with vanadium redox flow batteries (VRFB) leading the charge. These systems offer unlimited cycling capability and scalability, making them ideal for grid-stabilization in urban environments. Several pilot projects have shown 95% round-trip efficiency, significantly outperforming traditional storage methods.
Advanced control systems utilizing AI algorithms optimize these storage solutions in real-time, predicting demand patterns and managing energy distribution across the urban grid. This integration ensures maximum efficiency while maintaining grid stability, reducing energy waste by up to 30% compared to conventional systems.
Smart Buildings: The Energy Consumers of Tomorrow
IoT-Enabled Building Management Systems
By 2030, IoT-enabled Building Management Systems (BMS) will form the backbone of smart city infrastructure, transforming how buildings operate and interact with their occupants. These sophisticated systems leverage advanced sensors, artificial intelligence, and real-time data analytics to optimize energy consumption, enhance occupant comfort, and reduce operational costs.
Modern BMS platforms integrate multiple building functions, including HVAC, lighting, security, and access control, into a unified ecosystem. Smart sensors continuously monitor environmental conditions, occupancy patterns, and equipment performance, enabling predictive maintenance and automated adjustments. For instance, the Edge building in Amsterdam demonstrates how AI-driven systems can reduce energy consumption by up to 70% through intelligent climate control and daylight harvesting.
Energy optimization stands at the forefront of BMS capabilities. Advanced algorithms analyze historical usage patterns, weather forecasts, and real-time grid conditions to optimize building operations. Dynamic load management systems automatically adjust power consumption during peak demand periods, while smart meters enable granular monitoring of energy usage across different zones and systems.
The integration of renewable energy sources adds another layer of complexity to building management. Modern BMS platforms incorporate sophisticated energy storage management systems, coordinating between solar panels, battery storage, and grid power to maximize renewable energy utilization while minimizing costs.
Security and access control systems have evolved beyond simple card readers to include biometric authentication, AI-powered surveillance, and automated emergency response protocols. These systems not only enhance building security but also contribute to energy efficiency by controlling lighting and HVAC systems based on occupancy patterns.
Looking ahead, the next generation of BMS will incorporate digital twins and machine learning capabilities, enabling buildings to learn from occupant behavior and automatically adjust systems for optimal performance. This evolution towards truly intelligent buildings represents a crucial step in achieving the sustainability and efficiency goals of smart cities by 2030.
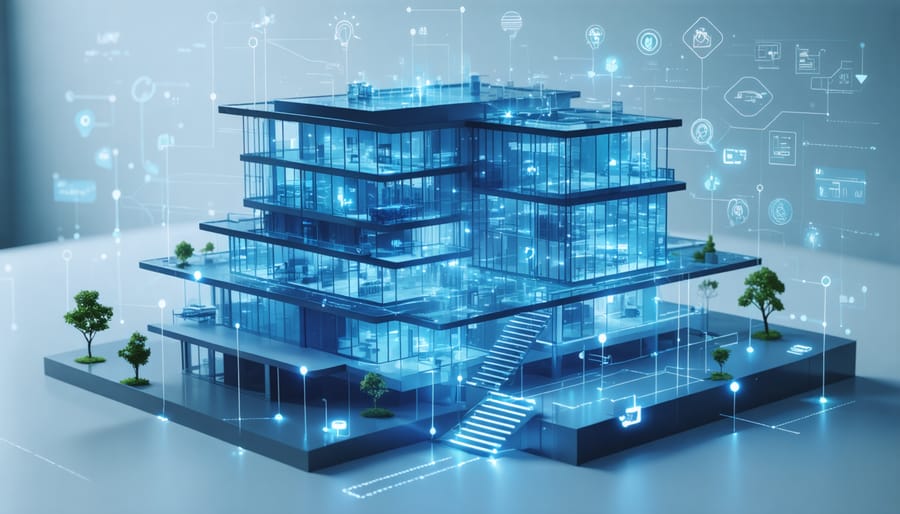
Demand Response and Dynamic Energy Pricing
In the evolving landscape of smart cities, demand response and dynamic energy pricing represent crucial mechanisms for optimizing energy consumption and distribution. These systems leverage real-time data analytics and automated grid management to balance supply and demand effectively while promoting sustainable energy usage patterns.
Smart meters and IoT sensors enable utilities to implement time-of-use pricing strategies, where energy costs fluctuate based on demand periods. During peak hours, prices increase to encourage reduced consumption, while off-peak rates incentivize users to shift their energy-intensive activities to periods of lower demand. This dynamic pricing model has shown remarkable success in pilot programs, with participating buildings reducing peak demand by up to 15-20%.
Advanced energy management systems in smart buildings automatically respond to price signals, adjusting HVAC operations, lighting systems, and other power-consuming equipment to optimize costs while maintaining occupant comfort. For example, the Amsterdam Smart City initiative demonstrated how commercial buildings could reduce energy costs by 30% through automated demand response systems.
Industrial facilities and large commercial complexes can participate in demand response programs, receiving financial incentives for reducing their power consumption during grid stress periods. These programs have proven particularly effective during extreme weather events or unexpected supply shortages, helping maintain grid stability and prevent blackouts.
The integration of renewable energy sources adds another dimension to dynamic pricing strategies. When renewable generation is high, prices typically decrease, encouraging consumption during these periods and reducing reliance on conventional power sources. This approach has been successfully implemented in Copenhagen, where smart grid systems coordinate energy consumption with wind power availability.
Looking toward 2030, artificial intelligence and machine learning algorithms will further enhance these systems, enabling predictive pricing models that anticipate demand patterns and optimize energy distribution across entire urban networks. This evolution in energy pricing and demand management will be fundamental to achieving the sustainability and efficiency goals of future smart cities.
Real-World Implementation Case Studies
Copenhagen stands as a prime example of successful smart energy implementation, having achieved remarkable progress toward its carbon neutrality goal by 2025. The city has integrated an intelligent grid system that manages renewable energy sources, with over 40% of its power now coming from wind energy. Real-time data monitoring allows for optimal distribution and storage of energy across the metropolitan area.
Singapore’s “Smart Nation” initiative demonstrates excellence in comprehensive urban energy management. The city-state has implemented a network of over 50,000 smart sensors monitoring everything from energy consumption to traffic patterns. Their Virtual Singapore platform creates a dynamic 3D city model that enables better energy planning and resource allocation.
Barcelona’s smart city transformation showcases innovative energy solutions through its smart street lighting system, which has resulted in 30% energy savings. The city’s smart grid project connects over 500,000 smart meters, providing real-time consumption data and enabling dynamic pricing strategies.
Amsterdam’s Smart City program has successfully implemented neighborhood-scale smart grid projects. The Nieuw-West district serves as a testing ground for innovative energy solutions, including virtual power plants and peer-to-peer energy trading. The project has achieved a 15% reduction in peak energy demand and increased renewable energy integration by 25%.
These implementations demonstrate that successful smart city energy systems require robust infrastructure, data-driven decision-making, and strong public-private partnerships. They also highlight the importance of scalable solutions that can be adapted to different urban contexts while maintaining efficiency and reliability.
As we approach 2030, the evolution of smart city energy systems represents a pivotal transformation in urban development. The integration of renewable energy sources, AI-driven grid management, and decentralized power distribution networks will fundamentally reshape how cities operate and serve their inhabitants. Industry leaders must recognize that success in implementing these systems requires a balanced approach combining technological innovation with practical infrastructure considerations.
The evidence from pioneering projects worldwide demonstrates that smart city energy solutions can reduce operational costs by 25-40% while significantly improving grid reliability and sustainability. However, the path forward demands careful attention to interoperability standards, cybersecurity protocols, and scalable implementation strategies.
Looking ahead, the construction industry must prepare for this transition by developing expertise in advanced energy management systems, sustainable building practices, and integrated urban planning. The successful realization of 2030 smart city goals will depend on collaborative efforts between construction professionals, technology providers, and urban planners, supported by forward-thinking policies and investment frameworks.
The future of urban energy systems lies in creating resilient, efficient, and sustainable infrastructure that can adapt to evolving city needs while maintaining optimal performance and reliability.